Quantum dots (QDs) and quantum rods (QRs) are tiny particles that emit specific colors of light. They are promising for various technologies, like advanced displays and quantum computing. To use them effectively, arranging them in precise patterns is crucial, but current methods are not efficient. Quantum rods, as the name suggests, are nano-sized structures that deviate from the dot’s circular form. Their elongated shape not only allows them to emit a spectrum of colors but also grants them the unique ability of controlling light polarization.
The challenge has always been to scale up quantum rods for practical applications while maintaining their desired alignment. MIT researchers have found an ingenious solution using DNA. The researchers harnessed the potential of DNA origami, a technique that uses the self-assembling properties of DNA molecules to create nano-sized structures with intricate designs. In this case, they fabricated origami structures with repeating diamond patterns, ingeniously placing quantum rods at key positions.
Essentially, the researchers have developed a fast and simple method to attach DNA molecules to QDs and QRs. They introduced these particles to a specific solution, then used ultrasound waves to create tiny bubbles in the mixture. By adding a special liquid, the DNA molecules efficiently stick to the particles. According to the study, published in Science Advances, this new approach created particles with densely packed DNA on their surfaces. This helps the particles stay stable in water-based solutions and ensures they can easily bind to other DNA structures. By precisely arranging QDs and QRs, the efficiency of LED displays can be improved, leading to brighter and longer-lasting screens.
One of the most promising aspects of this breakthrough is its scalability. Unlike conventional methods that often take days, this process wraps up within minutes. This speed and efficiency suggest that the technique could be seamlessly integrated into commercial systems. The MIT team envisions a future where wafers of quantum rods meticulously arranged on DNA scaffolds pave the way for a multitude of applications beyond even the realm of displays.
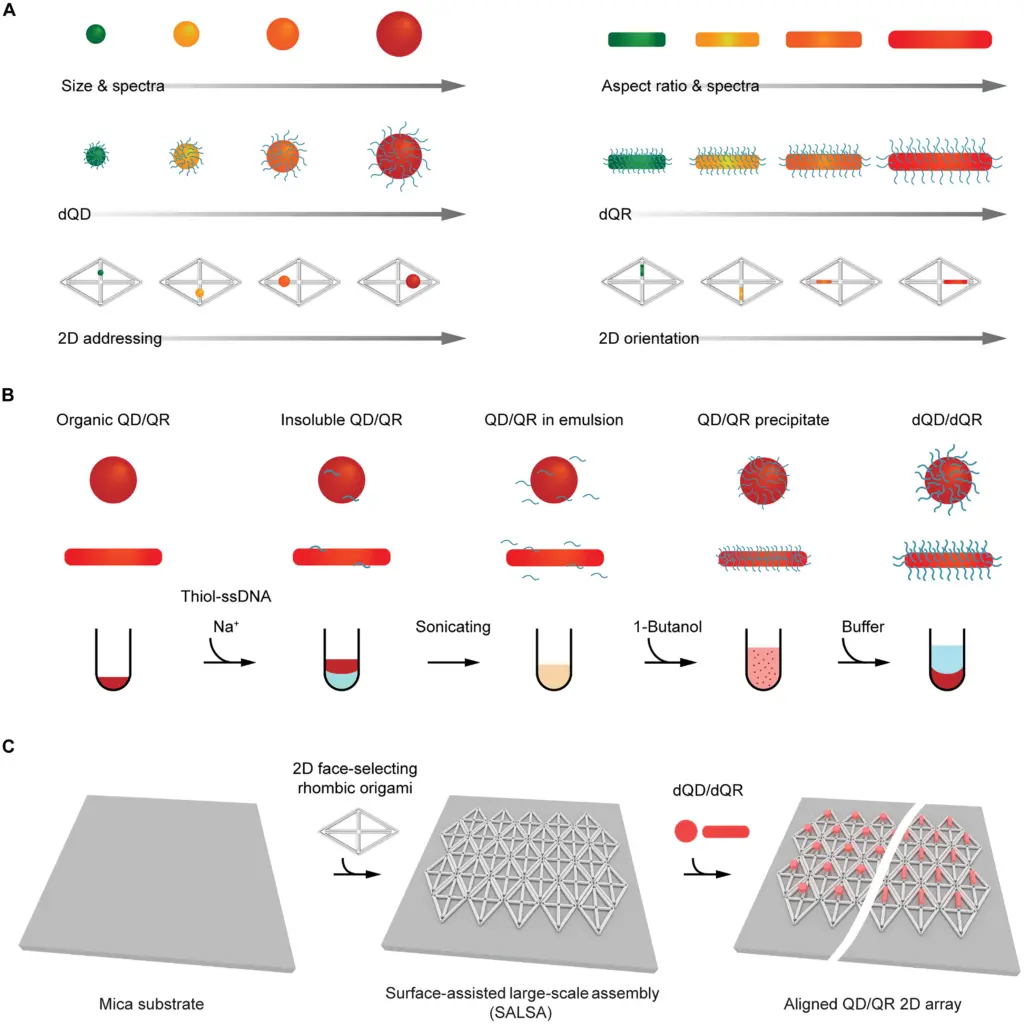
A) Dehydration-Assisted DNA Conjugation: This process involves efficiently attaching DNA molecules to QDs/QRs in their original organic solvent, transforming them into stable entities with high DNA density. The key steps include incubating QDs/QRs with thiol-derivatized single-stranded DNA (ssDNA) and using ultrasound to form an emulsion. The mixture is then dehydrated using 1-butanol, causing DNA to coat the QDs/QRs’ surfaces. Upon rehydration with an aqueous buffer, dQDs/dQRs with high-density DNA are formed.
B) Workflow for Preparing dQDs/dQRs: It involves steps like incubation, emulsion formation through ultrasound, dehydration with 1-butanol, and rehydration with an aqueous buffer. This process considerably reduces manufacturing time and simplifies the DNA functionalization of QDs/QRs.
C) Fabrication of Scalable QD/QR 2D Arrays: This part of the strategy focuses on fabricating scalable QD/QR 2D arrays with nanoscale spatial and orientational precision using SALSA and dQDs/dQRs. The concept involves assembling QD/QR-functionalized DNA origami structures onto solid substrates using the SALSA method. The DNA origami structures act as templates for organizing the QDs/QRs with controlled orientations and spacings. This process allows for precise arrangement and alignment of the QD/QR arrays, opening possibilities for advanced applications. (Source: Science Advances)
“The method that we describe in this paper is great because it provides good spatial and orientational control of how the quantum rods are positioned. The next steps are going to be making arrays that are more hierarchical, with programmed structure at many different length scales. The ability to control the sizes, shapes, and placement of these quantum rod arrays is a gateway to all sorts of different electronics applications,” says Robert Macfarlane, an associate professor of materials science and engineering.
“DNA is particularly attractive as a manufacturing material because it can be biologically produced, which is both scalable and sustainable, in line with the emerging U.S. bioeconomy. Translating this work toward commercial devices by solving several remaining bottlenecks, including switching to environmentally safe quantum rods, is what we’re focused on next,” adds Mark Bathe, an MIT professor of biological engineering and the senior author of the new study.
In addition to Macfarlane and Bathe, MIT postdocs Chi Chen and Xin Luo are the lead authors of the paper; Alexander Kaplan PhD ’23; and Moungi Bawendi, the Lester Wolfe Professor of Chemistry, are also authors of the study.
Reference
Chen, C., Luo, X., Kaplan, A. E. K., Bawendi, M. G., Macfarlane, R. J., & Bathe, M. (2023). Ultrafast dense DNA functionalization of quantum dots and rods for scalable 2D array fabrication with nanoscale precision. In Science Advances (Vol. 9, Issue 32, p. eadh8508). https://www.science.org/doi/abs/10.1126/sciadv.adh8508