I have to thank one of our subscribers, Refik Telhan, for bringing up the subject of metamerism. It made me think that it is probably good to get a primer on the subject matter. So, let’s start at the beginning. With your eyes and what you see.
As you sit comfortably watching your favorite show on TV, your eyes are at the receiving end of a sophisticated dance of photons that translates into the vivid imagery on the screen. Inside the TV, countless tiny LEDs work in concert, each shining in red, green, or blue. These primary colors blend in varying intensities right before your eyes to create the full palette of hues you perceive on the screen.
When this colorful light from the TV hits your eye, the light first passes through the clear outer layer of the eye, the cornea, which starts to focus it. The journey continues as the light is further bent by the lens behind your iris, finally projecting it toward the back of the eye, onto the retina.
Here, nestled within the layers of the retina, are the rods and cones—the photoreceptors that interpret light. The cones are the key players for the colors you’re soaking in from the TV. They come in three types, each one tuned to be sensitive to certain wavelengths of light corresponding to red, green, and blue. Red hues, for example, rouse the L cones more than the M or S cones, while blues preferentially stir the S cones. The green tones strike a chord with the M cones.
In the brain’s visual centers, this information is deftly processed. It’s not just the individual stimulation of cones that the brain looks at; it’s the entire ensemble—how much each type of cone is stimulated in relation to the others. The brain interprets these signals, weaves them together, and creates the sensation of seeing colors. It’s a process that allows you to perceive the full spectrum of colors that make up the image on the TV.
Moreover, this isn’t happening in isolation. The brain is also interpreting context, comparing the colors on the screen with those around it and adjusting your perception dynamically based on lighting and the colors themselves. This context can make the same color appear different under different circumstances, all thanks to the brain’s interpretation.
As you gaze at the screen, your rods are taking a back seat, as they’re more attuned to detecting brightness levels in low-light conditions, where color is less discernible. In the well-lit living room, it’s the cones that are basking in the light show from your TV, allowing you to appreciate the full brilliance of colors on display.
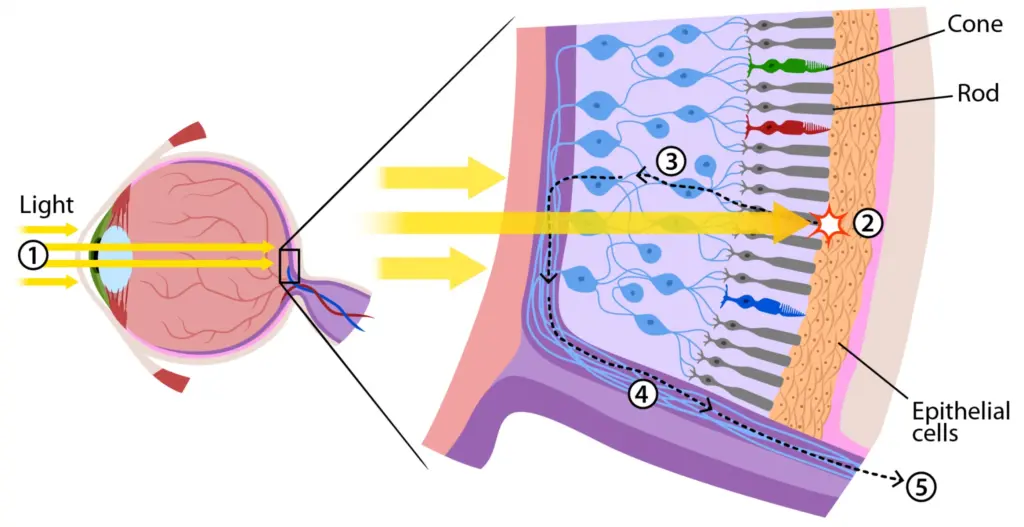
On a more technical note, the process of perceiving light from a TV is an intricate symphony of optical physics and neurobiology. The LEDs are semiconductors that emit light when an electric current passes through them, a phenomenon known as electroluminescence. The color and brightness of this light are controlled by the material and engineering of the diodes, which are designed to produce the three primary colors at different intensities. When these lights reach the eye, they encounter the retina’s phototransduction pathway, where photopigments within the cones undergo a molecular change upon absorbing photons, leading to a cascade of chemical reactions that ultimately generate a nerve impulse. This signal is modulated by various retinal cells, including bipolar and ganglion cells, before being relayed to the brain. The brain then interprets these impulses, contrasting and blending them, to form the detailed, colorful picture you enjoy. This visual processing includes not only the raw data of hue but also the interpretation of contrast, depth, and movement, creating a cohesive visual experience from the pulsating lights of the LED display.
The Motion of the Waves
Varying wavelengths of light have profound impacts on the perception of color, depth, and even the physiological and psychological state of an individual. In terms of color perception, each wavelength within the visible spectrum corresponds to a different perceived color. Short wavelengths are seen as blue or violet, middle wavelengths as greens, and long wavelengths as reds. The human eye’s cones are differentially sensitive to these wavelengths, with S-cones responding to short wavelengths (blue light), M-cones to medium wavelengths (green light), and L-cones to long wavelengths (red light). The brain interprets the varying degrees of stimulation across these three types of cones to perceive the full range of colors in our environment.
Moreover, wavelength affects the perception of saturation and brightness. Shorter wavelengths, such as blue light, tend to scatter more within the eye’s media, leading to a less sharp image under certain conditions, which is why distant objects often appear bluish (Rayleigh scattering). Conversely, longer wavelengths like red and yellow are less scattered and can contribute to sharper vision.
Beyond color perception, light wavelengths can also have other impacts. For instance, blue light, especially at shorter wavelengths, can be more stimulating and is known to suppress the production of melatonin, the hormone responsible for regulating sleep, thus potentially impacting circadian rhythms and sleep patterns. In contrast, warmer lights with longer wavelengths tend to have a calming effect and are less likely to interfere with sleep.
In terms of psychological effects, certain wavelengths can influence mood and cognitive performance. Cooler light, which has a higher proportion of blue wavelengths, is often associated with alertness and improved performance, while warmer tones can create a sense of relaxation. Retail spaces, workplaces, and educational environments often utilize this knowledge to enhance customer experience, productivity, or learning outcomes.
In art and design, the manipulation of light and its wavelengths can alter the perceived texture and quality of materials. Artists and designers think carefully about the light under which their work will be viewed, understanding that the color temperature and wavelength of the light can dramatically affect the colors and the emotional response to their work.
The Phenomenon of Metamerism
Metamerism is a phenomenon where two objects with different spectral power distributions appear matched in color under one light source, but when illuminated by a different light source, mismatches become visible. As we just learned, the human visual system works by integrating light across three broad bands of wavelengths corresponding to our cone photoreceptors. Many different spectral combinations can produce the same triplet of cone responses and be perceived as identical colors.
However, if the light source changes, the relative amounts of light at different wavelengths changes. This alters the cone responses and can reveal color differences between two objects that previously matched. So, metamerism reveals the trichromatic nature of human color vision – we can’t discriminate all possible spectral combinations.
The degree of metamerism depends on the observer and the illuminant. Individuals vary in their color matching functions. It is most noticeable between objects with narrowband spectral reflectances versus broad reflectances. Two pure monochromatic colors will always appear matched. And, it poses challenges for color reproduction, as a color match under one light may not hold in a different environment.
Narrowband spectral reflectances refer to materials or objects that reflect only a narrow band of wavelengths of light, rather than reflecting broadly across the visible spectrum. They exhibit a spiky reflectance spectrum with a narrow peak at a particular wavelength, and very low reflectance at other wavelengths. Common examples are lasers, LEDs, and fluorescent materials that emit specific pure colors. Dyes and pigments can also be engineered to have narrowband absorption spectra, resulting in narrowband reflection. Natural examples are rare, but some gemstones, minerals, and bird feathers can produce very spiky reflectance spectra. In plants, the reflectance spectrum from chlorophyll absorption causes a narrow peak in the green region.
Two materials with narrowband reflectances may match in color under one illuminant, but small shifts in illumination can reveal very different spectral makeup. This makes narrowband reflectances more prone to metamerism effects compared to broad, smooth spectral curves. LEDs inherently produce light in a relatively narrow band of wavelengths, so by their nature they do not have a broad, smooth reflectance spectra.
However, there are a few ways LEDs can be engineered to approximate a broader spectral output:
- Phosphor-converted LEDs – A blue LED is used to excite a yellow phosphor, which combines to produce a broader band white light. This can approximate a smooth spectrum but there are still peaks at the blue and yellow wavelengths.
- Multi-chip LEDs – Combining the outputs of red, green, and blue LED chips together can produce a fuller spectrum, but there will still be spikes from each LED.
- Quantum dot LEDs – Using quantum dots as the phosphor can enable finer tuning of the wavelengths emitted, producing a fuller spectrum. But there are still emission peaks.
- Stacked/Cascade LEDs – Thin multi-layer LED structures allow more spectral tuning by manipulating the modes and combinations of overlaid emitters.
Widening Color Gamuts and Metamerism
Widening the color gamut is often marketed as an unconditional improvement, when in reality there are downsides like increased metamerism that need to be considered. More education is needed so consumers understand this.
For professional use cases like color-critical work, metamerism is a major concern. Displays optimized for wide gamuts may not be suitable. Selecting displays that prioritize color accuracy over wide gamuts could be preferable. However, there is no one-size-fits-all solution. The needs of casual viewers watching movies may be different from graphic designers collaborating on a project. Each use case needs to weigh the pros and cons of wide gamuts based on their specific requirements.
While complete elimination of metamerism may not be feasible, steps could be taken to minimize it through thoughtful display design and characterization. Providing detailed specs on a display’s metameric properties can help inform professionals. Though there are challenges in how to communicate this clearly to non-experts.
But, putting aside all of the inherent inconsistencies in what constitutes color in displays, wider color gamuts get displays closer to rendering real-world colors, and that seems to be biggest selling point of their pursuit. That and the fact that it sounds great on a marketing sheet. Yet, wider color gamuts are also, inherently, designed to allow for the likelihood that content produced in HDR or to DCI-P3 standards for cinema can be enabled on displays.
Now you have the ins and outs of metamerism. It may not mean much to the average consumer, although I am pretty sure that if you educated a user about the subject and they understood how metamerism functions, they would probably be better able to recognize it, and then it would be a problem. It is definitely an issue for professional users who rely on the absolute accuracy of color in their displays, but I guess they are a small enough niche that no one is bothered if they make a little noise about the matter. And, at the end of the day, the movie experience is what sells displays, and that means there is a small audience of Hollywood creatives who really drive the color quality argument for displays. Obviously, enabling wider color gamuts is about widening the net to capture every nuance of color that is programmed into a digital movies stream.
Ironically, even in the cinema, movie theaters use various types of projectors – Xenon lamps, LED, lasers etc. Each has their own spectral power distribution. If a movie is color graded for one type of projector, the colors may not match well when shown using a projector with a different spectrum. So, common standard illuminants used in production may not match the theater’s projection system, increasing metameric issues. Theaters also have relatively uncontrolled lighting conditions – ambient light spectra can differ across seats/screens and viewers have different individual color vision and perception, leading to increased observer metamerism. And who doesn’t like those new reclining loungers in movie theaters? Good, but a change in seating angle relative to the screen shifts the colors seen due to properties of the projection surface.
We pay for perfection in our displays but they should come with a warning: nothing is what it appears to be.